Steps in the Evolution of Scientific Naturalism
Introduction
In the quest to decipher the universe, humanity has navigated from the arcane geometries of Pythagoras to the mind-bending theories of particle physics and cosmology, each step an echo in our ceaseless search for reality. As we stand on the cusp of understanding the fundamental particles that dance to the tune of nature’s laws and the cosmic ballet that has unfolded over billions of years, the philosophy of scientific naturalism has emerged as the intellectual scaffolding upon which we construct our modern worldview. Yet, as we delve deeper into the subatomic world and peer farther into the cosmic abyss, we are compelled to confront not just the outer limits of our scientific understanding but also the boundaries of what can be known, challenging us to reconcile our profound discoveries with the existential questions that have gripped humanity since the dawn of conscious thought.
Long before the rigour of scientific inquiry shaped our understanding of the cosmos, early human societies grappled with the inexplicable through the lens of animism. In the boundless stretches of forests and the open savannas, hunter-gatherers saw spirits in every rock, river, and gust of wind—a universe teeming with intent and agency. As the wheel of time turned, these rudimentary beliefs merged into the pantheons that presided over the earliest empires. Mighty gods and goddesses were invoked to explain phenomena beyond human control, from the harvest’s bounty to the destructive wrath of natural disasters. These mythological frameworks served as the scaffolding upon which civilisations were built, offering both explanation and solace. Yet, they were limited by the empirical knowledge of their times.
Classical Greek Philosophy – the Seeds of Modern Western Thought
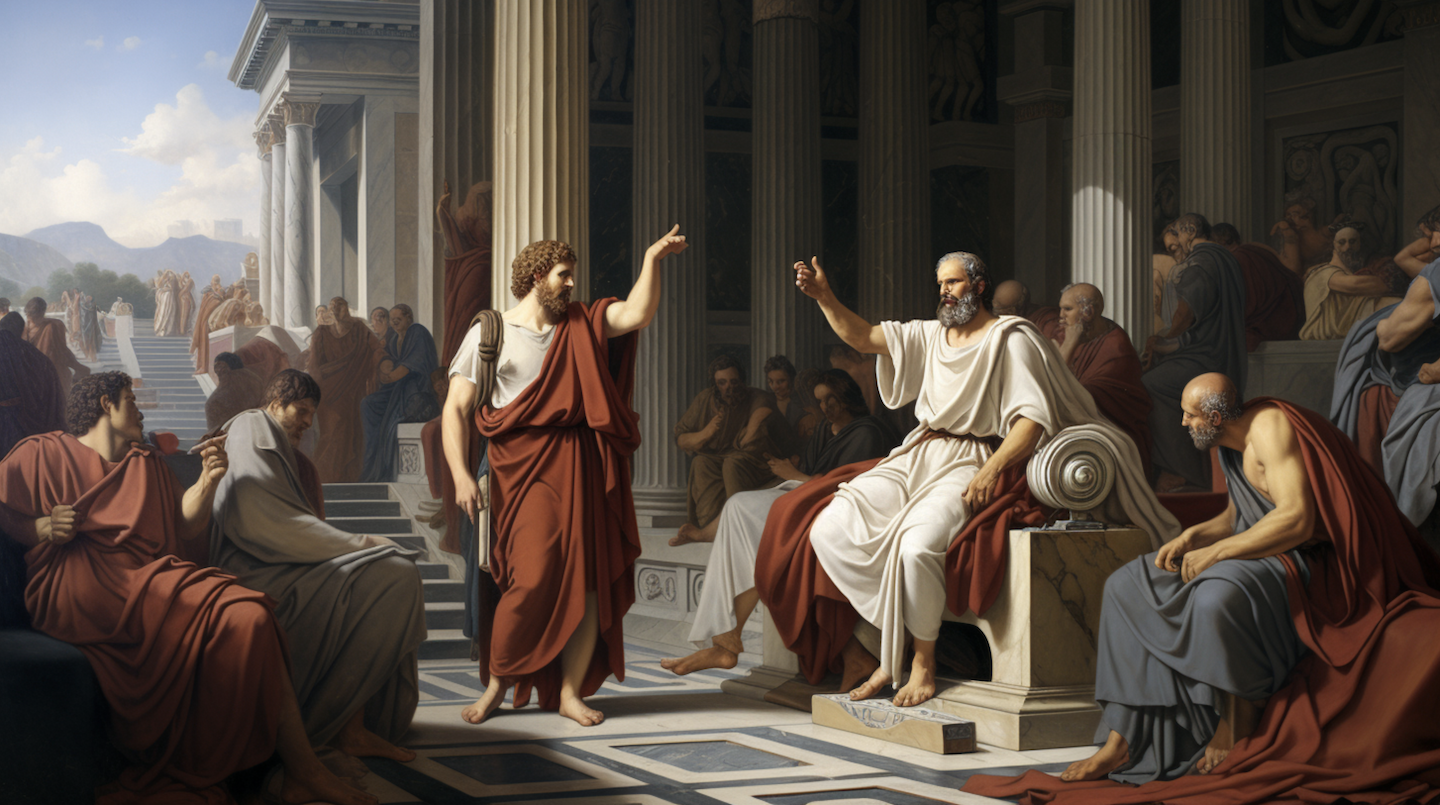
In the fertile intellectual landscape of ancient Greece, a shift from myth to reason marked the birth of Western philosophy. Among the pioneers was Thales of Miletus, who stepped away from mythological attributions to natural phenomena and declared water as the primordial ‘arche,’ or principle, underpinning all things. This was a seminal moment, as Thales substituted empirical observation for divine explanation, instigating a philosophical tradition that valued observation and reason.
No less significant was Pythagoras, whose mysticism was grounded in mathematical rigour. In Pythagorean thought, the cosmos was arranged according to harmonic ratios, an idea encapsulated in the notion that ‘all is number.’ While veering into the metaphysical, Pythagoras nonetheless instituted the idea that reality had an underlying, discoverable order—introducing an abstract mathematical language that centuries later would become the bedrock of scientific inquiry.
Pre-Socratic thinkers, diverse as they were, continued this intense scrutiny of reality’s fabric. While Heraclitus considered change the only constant, captured poignantly in his allegory of the ever-changing river, Parmenides set forth an unchanging, eternal essence as the real substratum of the world. Democritus, meanwhile, posited a theory of atomism, envisioning a universe made of indivisible particles in constant motion. Though empirically nascent, this theory would astonishingly echo through millennia to resonate with our contemporary understanding of matter.
From Thales’ elemental reasoning to Pythagoras’ abstract harmonics, and through the Pre-Socratics’ varied ontological proposals, each thinker contributed a unique lens through which to view the enigma of existence. These conflicting yet transformative ideas did not simply displace the mythologies of yore. Still, they laid the groundwork for an enduring philosophical and scientific legacy, inviting humanity to probe ever deeper into the nature of reality.
The luminescence of Athenian philosophical inquiry reached an apex in the works of Plato and his student Aristotle, whose ideas collectively served as intellectual cornerstones for Western civilisation. Plato, deeply influenced by Socratic dialogues, extended the philosophical enterprise into metaphysics with his Theory of Forms. According to Plato, the empirical world was a shadowy projection of a higher, ideal realm. The Forms—a perfect triangle, an ideal of justice—existed as eternally true concepts, accessible only through rigorous intellectual labour. Plato’s metaphysical framework laid the groundwork for subsequent religious and philosophical traditions that sought to transcend the material world in pursuit of ultimate truths.
Aristotle diverged dramatically from his teacher, opting for an empirically grounded approach to understanding reality. His meticulous observations led to the development of formalised logic and classifications that sought to explain the natural world in terms of ‘causality.’ His four causes—material, formal, efficient, and final—offered a comprehensive explanation for why things are as they are, integrating both the substance and the purpose of objects and phenomena. Despite its limitations exposed by modern science, Aristotle’s natural philosophy dominated scholastic thought. It was deeply integrated into Christian, Islamic, and Jewish intellectual traditions.
The debate between Plato’s idealism and Aristotle’s empiricism crafted a dual legacy that would frame European thought for over a millennium and a half. Their enduring ideas served as both a fulcrum and a point of departure for thinkers who followed, leaving an indelible imprint on humanity’s ceaseless quest to understand the very sinews of reality.
From Copernicus to the Age of Revolution: Shifting the Cosmic Perspective
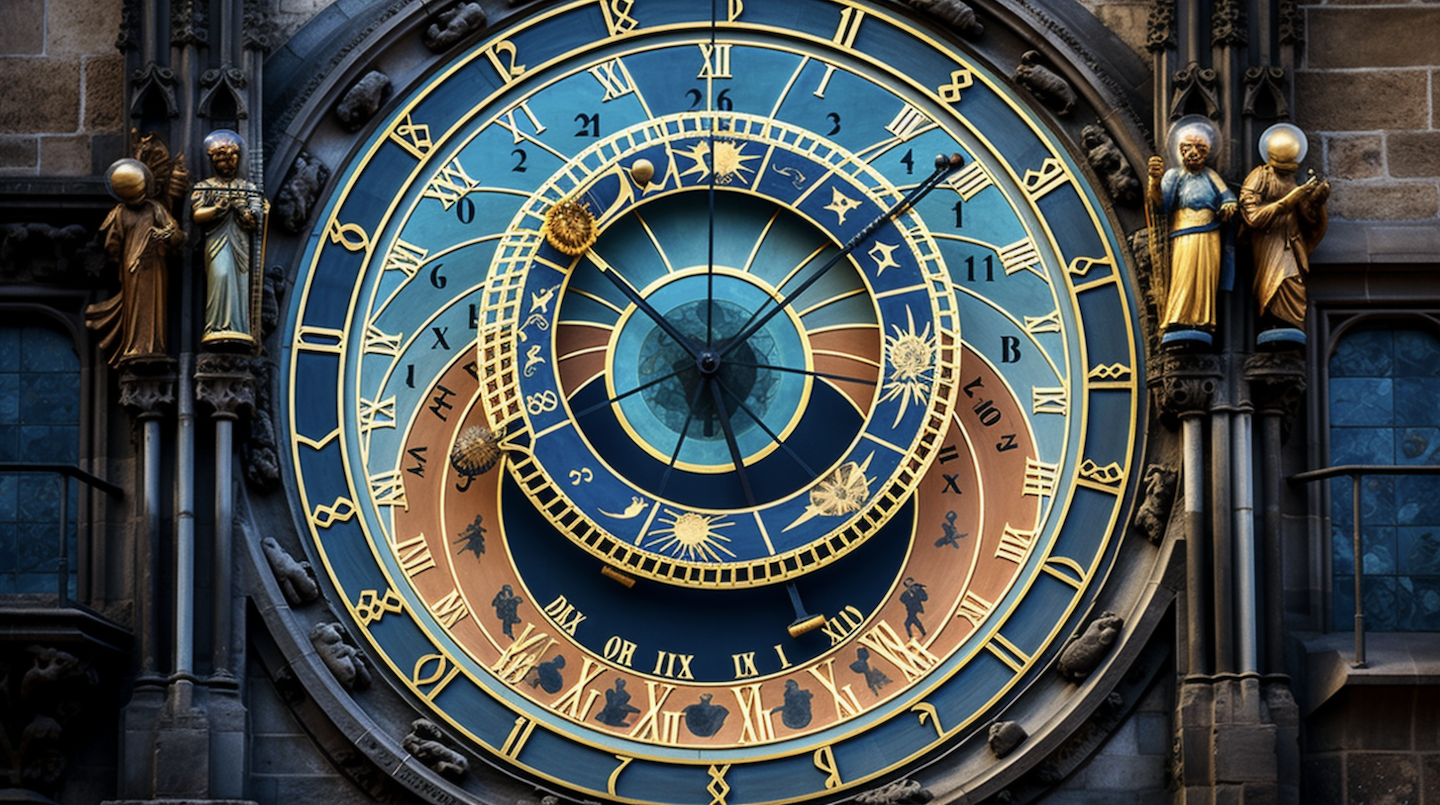
The onset of the Renaissance ignited a transformative period in which long-held geocentric views of the universe were overturned, and humanity’s place within the cosmos was reevaluated. At the forefront of this intellectual upheaval was Nicolaus Copernicus. Daring to challenge the Ptolemaic model that had held sway for over a millennium, Copernicus proposed a heliocentric universe with the Sun—not the Earth—as its centre. Though revolutionary, his ideas found limited acceptance during his lifetime, mainly because they were mathematically complex and lacked empirical validation.
It took the combined efforts of Johannes Kepler and Galileo Galilei to elevate Copernicanism from an elegant mathematical exercise to empirical reality. Kepler refined the model by introducing elliptical orbits, resolving observational discrepancies that had dogged Copernican circles. At about the same time, Galileo’s telescopic observations revealed celestial phenomena—like the moons of Jupiter and the phases of Venus—that could only be reconciled within a heliocentric framework.
The shift from a geocentric to a heliocentric model was more than a mere adjustment of astronomical parameters; it was a paradigm shift reverberating through the corridors of philosophy, religion, and society. Copernicus, Kepler, and Galileo collectively shattered the crystalline spheres of medieval cosmology, making way for a universe governed by mathematical laws and open to empirical investigation. The Copernican Revolution did not merely alter our understanding of the celestial dance; it catalysed a scientific and intellectual awakening that would ultimately sow the seeds for the Age of Revolutions.
While the Copernican Revolution shattered celestial illusions, René Descartes ventured inward, radically redefining our understanding of knowledge, mind, and reality. Known for his seminal phrase, ‘Cogito, ergo sum’ — ‘I think, therefore I am’—Descartes sought to establish a reliable foundation for scientific and philosophical inquiry. Discontented with sensory perception and empirical experience, susceptible to doubt, he delved into the domain of thought as the only irrefutable proof of existence.
Descartes’ approach was dualistic, delineating between res cogitans, the realm of the mind, and res extensa, the realm of extended, physical matter. This Cartesian dualism would profoundly influence the study of metaphysics, epistemology, and the philosophy of mind, spurring debates that still resonate today. The dualistic framework also provided an intellectual space for science to flourish, separate from theology and metaphysics. By allocating the physical world to the domain of mathematical analysis and empirical investigation, Descartes set the stage for the Scientific Revolution and, eventually, for the Enlightenment.
Notwithstanding the persistent critiques of his dualism—seen by some as creating an unbridgeable chasm between mind and matter—Descartes’ impact remains indelible. His method of doubt, his coordination system in geometry, and his mechanistic view of the physical world have all been instrumental in shaping the subsequent evolution of science and philosophy. His philosophical edifice did more than answer questions; it taught us how to question the very fabric of reality, foregrounding the power of rational scepticism in our ceaseless human quest for understanding.
Isaac Newton, the towering intellect of the 17th century, synthesised the advancements of his predecessors into a cohesive framework that seemed to unlock the universe’s secrets. His formulation of the laws of motion and universal gravitation offered an unprecedentedly coherent and quantifiable explanation for both terrestrial and celestial phenomena. From the fall of an apple to the orbit of planets, the universe appeared to be a grand machine, functioning according to immutable laws that could be articulated mathematically.
Newton’s mechanistic conception of the universe had profound implications, serving as a philosophical bedrock for a deterministic worldview. If all matter abided by fixed laws, it seemed logical to assume that the universe’s future and past could, in principle, be calculated from its present state. This clockwork universe left little room for randomness or divine intervention, challenging long-standing theological perspectives and creating a schism that would persist for centuries.
Though Newton harboured deep religious convictions and even engaged in alchemical practices, his work laid the scientific foundation for a universe governed solely by natural laws. His ‘Principia Mathematica’ was more than a seminal text in physics; it was an intellectual milestone that empowered humanity with the conviction that rational inquiry and empirical observation could decode the cosmos. In effect, Newton’s laws set the stage for the Enlightenment, where reason would be heralded as the ultimate arbiter in our ongoing quest to understand the complex tapestry of reality.
The Enlightenment era was marked by an efflorescence of intellectual inquiry spurred by a newfound faith in human reason and its capacity to illuminate the contours of reality. Among its leading lights was Immanuel Kant, a polymath whose work stands as a fulcrum of modern philosophy. Kant sought to reconcile the burgeoning empirical sciences with moral and metaphysical concerns. His ethical framework of ‘categorical imperatives’ introduced a system of moral universalism based on rationalism, asserting that moral principles should apply universally, irrespective of cultural or individual differences.
Contrary to the mechanistic determinism suggested by Newtonian physics, Kant also emphasised the concept of free will. While acknowledging the predictive power of scientific laws in the realm of phenomena, he argued that the realm of ‘noumena’—the things-in-themselves—lay beyond the purview of human understanding. This dual perspective granted room for ethical freedom within a causally determined universe.
Kant was not alone; Enlightenment thought was a tapestry woven from the contributions of many, from Voltaire’s advocacy for civil liberties to Adam Smith’s economic theories. The Enlightenment’s intellectual hubris—that reason and science alone could define reality and human nature—found its political manifestation in the American and French Revolutions. These revolutions, ignited by Enlightenment ideals, sought to construct new social and political realities rooted in the principles of liberty, equality, and fraternity.
Yet, the revolutions also unveiled the complexities and contradictions inherent in Enlightenment thought. While the American Revolution led to a new republic and the codification of democratic ideals, the French Revolution spiralled into the Reign of Terror, exposing the perils of ideological zeal detached from human complexities.
The Enlightenment was both a philosophical watershed and a cautionary tale. It empowered humanity with the tools of reason and science but also laid bare the limits of understanding a multifaceted reality solely through a rationalist lens. The era forever changed the trajectory of humanity’s quest for understanding, serving as both a lighthouse and a cliff in our ever-evolving narrative of reality.
From Steam Power to String Theory: Reshaping our World.
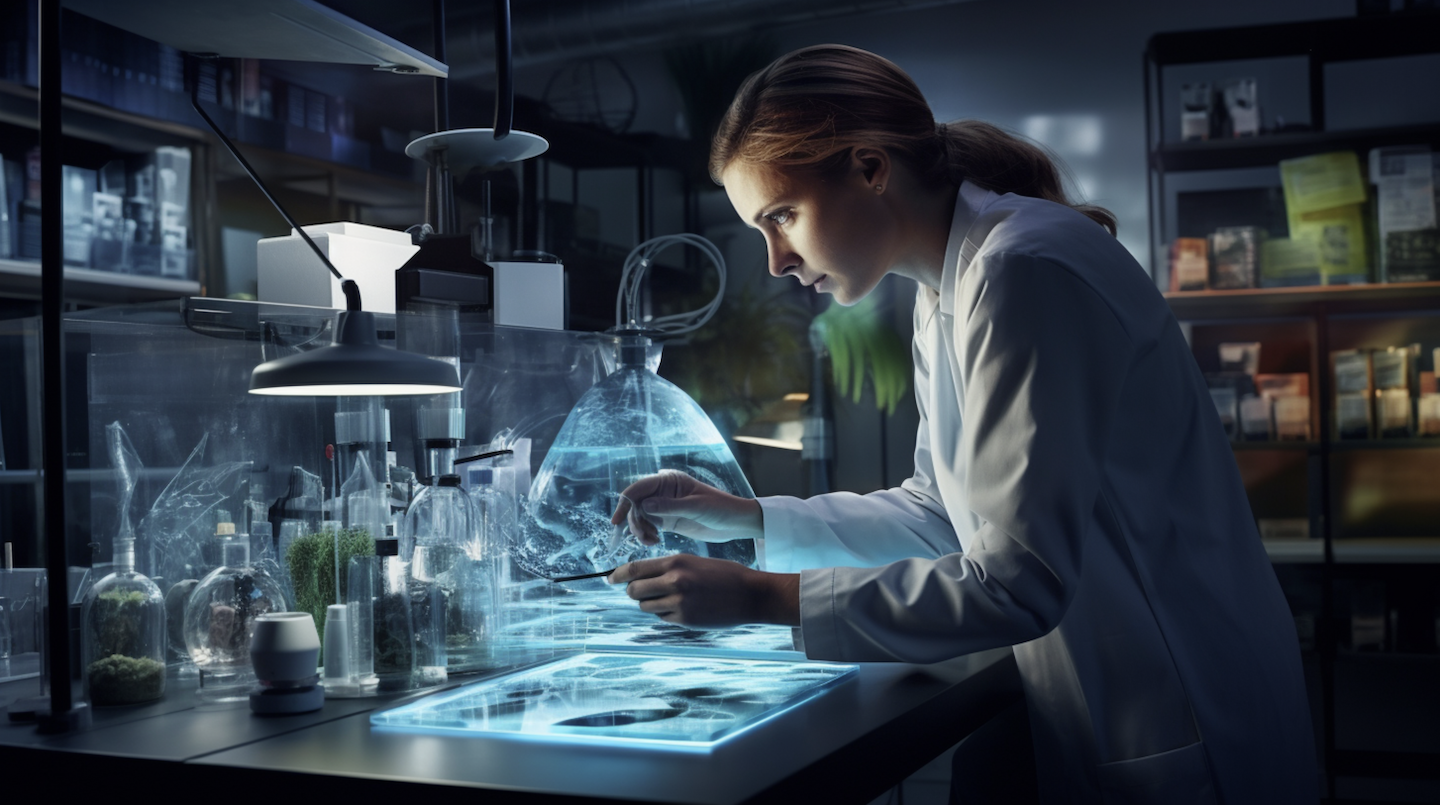
As humanity’s intellectual pursuits advanced, a new marvel of understanding unfolded in the form of thermodynamics—the science of energy, heat, work, and their interrelations. Pioneered by figures like Sadi Carnot, Lord Kelvin, and James Clerk Maxwell, thermodynamics offered a set of universal laws governing mechanical systems and natural phenomena ranging from chemical reactions to biological processes. At its core, the First and Second Laws of Thermodynamics defined the conservation of energy and the inescapable increase in entropy, or disorder, within closed systems.
This newfound comprehension of energy conversion catalysed the Industrial Revolution, enabling the transformation of coal and steam into mechanical work. Suddenly, trains could traverse continents, factories could mass-produce goods, and energy could be harnessed for myriad human needs. Yet, the implications extended far beyond the industrial realm. Thermodynamics influenced various scientific disciplines, providing a language that could describe phase transitions, power cycles, and even the behaviour of black holes.
The laws of thermodynamics also introduced limits on our understanding of the universe. With its decree that entropy must increase, the Second Law imposed boundaries on perpetual motion and perfect efficiency, laying the foundation for existential questions about the universe’s ultimate fate. The notion of irreversible processes, a corollary to increasing entropy, emerged as a poignant reminder of time’s unidirectional march.
Thermodynamics stood as a testament to the potency of scientific laws in describing diverse phenomena, cementing its place as a cornerstone in our ever-expanding edifice of reality. As both a practical tool and a philosophical concept, thermodynamics became a linchpin, connecting disparate domains of science and deepening our understanding of the natural world.
Parallel to the industrial and scientific advancements rapidly reshaping the human landscape, a quiet revolution was stirring in biology. Charles Darwin’s ‘On the Origin of Species’ burst onto the scene in 1859, forever altering our understanding of life. Going against the prevailing notion of a static, unchanging biosphere, Darwin introduced the radical idea of evolution by natural selection. It seemed life was not a fixed tableau but a dynamic, ever-changing tapestry woven from the threads of variation, selection, and inheritance.
Darwin’s theory offered a cohesive and empirically grounded explanation for the baffling diversity of life on Earth. Species were not immutable archetypes; they were flexible populations moulded by environmental pressures over vast time scales. This shift in perspective extended beyond biology, impacting social sciences, ethics, and even theology, challenging long-held assumptions about human nature and our place in the cosmos.
The evolutionary paradigm provided a stark counterpoint to Newtonian physics’s deterministic, mechanistic universe. In the Darwinian world, randomness and contingency played critical roles, creating a nuanced interplay between determinism and chance. While thermodynamics described a universe marching inexorably towards higher entropy, evolutionary biology depicted a biosphere bursting with complexity and innovation, forged through countless iterations of trial and error.
Darwin’s theory of evolution not only redefined the biological sciences but also offered a profound metaphysical narrative. Life was no longer the result of divine fiat but a magnificent unfolding, where simplicity begets complexity and where a cornucopia of forms and functions emerged from the primordial soup, each intricately adapted to its niche. The Origin of Species became more than a scientific treatise; it became a seminal chapter in humanity’s ever-growing tome of reality.
Even as Darwin reshaped our understanding of life’s diversity, another intellectual titan, James Clerk Maxwell, was about to redefine our comprehension of fundamental forces. Published in the early 1860s, Maxwell’s ‘A Treatise on Electricity and Magnetism’ achieved something monumental: the unification of electricity and magnetism into a single, coherent theory of electromagnetism. Through a set of elegant mathematical equations, Maxwell described how electric and magnetic fields were interlinked, propagating through space as electromagnetic waves.
Maxwell’s work transcended mere description. The abstract concept of a ‘field’ gained prominence in the scientific lexicon for the first time. Fields became the invisible scaffolds upon which particles and forces interacted, fundamentally altering our view of empty space as passive and inert. Now, space was an active participant, teeming with fields that determined the behaviour of matter and energy.
The practical applications of Maxwell’s equations were revolutionary. They became the theoretical bedrock for various technologies defining modern life, from radio and television to radar and wireless communication. Moreover, they set the stage for the advent of quantum mechanics and Einstein’s theory of general relativity, each of which would later refine and expand upon the concept of fields.
Yet, the treatise did more than just lay the groundwork for technological progress. It served as a symbolic unification, mirroring the era’s broader scientific quest to unite disparate phenomena under universal laws. Just as Darwin’s theory synthesised the tapestry of life and thermodynamics laid out the inescapable rules of energy and heat, Maxwell’s equations tied together the once-disparate realms of electricity and magnetism, pointing to an underlying simplicity beneath the apparent complexities of the universe.
In short, Maxwell didn’t just rewrite the book on electromagnetism; he offered a new chapter in humanity’s grand narrative, reinforcing the awe-inspiring notion that the universe is not a chaotic cacophony but a symphonic masterpiece waiting to be deciphered by the inquiring mind.
At the cusp of the 20th century, another series of revelations shook the foundations of our understanding of matter and energy. In 1895, Wilhelm Conrad Roentgen discovered X-rays, mysterious waves capable of penetrating solid objects, inaugurating a new era of medical diagnostics. Not long after, Marie and Pierre Curie isolated radium and polonium, introducing the world to the enigmatic phenomena of radioactivity.
These discoveries were not merely scientific curiosities; they had immediate and transformative applications. Roentgen’s X-rays revolutionised medical science, allowing doctors to peer into the human body without invasive procedures. The Curies’ work on radioactivity led to innovations in fields ranging from medicine, where radioactive isotopes became invaluable tools for treatment and diagnosis, to energy, laying the groundwork for nuclear technology.
However, the implications of these discoveries extended far beyond their practical uses. Building on the Curies’ pioneering research, Ernest Rutherford elucidated the atom’s structure, revealing it to be a miniature solar system of sorts, with electrons orbiting a dense nucleus. This model replaced long-standing views of atoms as indivisible units of matter, suggesting instead a complex internal structure replete with subatomic particles.
Rutherford’s atomic model radically altered our understanding of matter itself. Atoms were no longer the fundamental, unbreakable components of the universe but were complex entities subject to their own rules and behaviours. This realisation opened up a new frontier in scientific inquiry, setting the stage for quantum mechanics and, eventually, the standard model of particle physics.
Together, these discoveries in radiation and atomic structure exemplified the duality of scientific progress: they solved practical problems and improved human well-being while forcing us to revise our conceptual frameworks. Just like thermodynamics reconciled the laws of heat, work, and energy, and Maxwell’s equations unified electric and magnetic phenomena, the insights of Roentgen, the Curies, and Rutherford presented a fresh synthesis, knitting together the worlds of the immensely small and the humanly relevant. In doing so, they added yet another layer to our continually evolving tapestry of reality.
Shortly into the 20th century, a patent clerk named Albert Einstein upended the laws of physics, triggering a paradigm shift whose repercussions are still felt today. In 1905, Einstein unveiled his theory of Special Relativity, which posited that the laws of physics are the same for all observers, regardless of their motion. But this was not a mere refinement of existing ideas; it was a demolition of the intuitive, Newtonian universe. Suddenly, time and space were not independent entities but interwoven into a four-dimensional spacetime continuum.
Then, in 1915, Einstein went further with the theory of General Relativity, which described how massive objects like stars and planets curved the spacetime around them, creating what we perceive as gravity. This replaced the Newtonian concept of gravitational attraction as a force acting over a distance, with a geometric theory that required no such ‘action at a distance.’
Einstein’s theories were transformative, not just in the abstract realm of physics but in practical, measurable ways. General relativity refined our understanding of planetary motion and timekeeping, laying the conceptual groundwork for technologies ranging from GPS to black hole imaging. At a fundamental level, the theory of relativity replaced the deterministic, clockwork universe with one far more strange and malleable, where time dilates, space curves, and mass and energy are interchangeable.
More philosophically, Einstein’s work shifted the human narrative on reality. Our universe was no longer the rigid, linear stage described by Newtonian mechanics, but a dynamic, bending landscape influenced by matter and energy. It suggested a cosmos not only strange and counterintuitive but also elegant and unified, governed by laws accessible through human reason and ingenuity.
Einstein didn’t merely change physics; he changed how humanity perceives its place in the cosmos. For the first time, we began to see the universe not as a static backdrop but as an ever-changing, interconnected tapestry of phenomena, bound by principles as beautiful as they are confounding. In this, Einstein crystallised a theme that runs like a golden thread through the annals of scientific discovery: the universe is not merely to be observed and described, but to be understood in its profound, majestic complexity.
Hot on the heels of Einstein’s universe-altering theories came another scientific upheaval that shattered the very framework of classical physics and took us into the realm of the subatomic—Quantum Mechanics. Initiated by the work of Max Planck and later formulated by Werner Heisenberg, Erwin Schrödinger, and Niels Bohr among others, quantum mechanics confronts us with a reality far removed from our everyday experiences and intuitions.
Planck’s quantum hypothesis, which explained the radiation from a black body, suggested that energy is emitted in discrete packets or ‘quanta,’ demolishing the continuity of classical physics. Heisenberg’s Uncertainty Principle further rocked the boat by asserting that simultaneously, it is fundamentally impossible to precisely measure specific pairs of properties, like position and momentum. This was not a mere limitation of our measurement tools but an intrinsic feature of the universe itself.
Schrödinger’s equation provided a probabilistic framework for predicting the behaviour of particles, yet also left us with paradoxes like his famous ‘Schrödinger’s Cat’ thought experiment. It forced us to contemplate a reality where a cat could be simultaneously alive and dead, undetermined until observed, highlighting the mysteries and paradoxes that define quantum behaviour.
These quantum phenomena introduced a new wave-particle duality, suggesting that particles like electrons could demonstrate characteristics of both particles and waves. Suddenly, the universe at its most fundamental level was governed not by deterministic laws but by probabilities and uncertainties. Newton’s deterministic, clockwork universe and even the curved spacetime of Einstein gave way to a realm of inherent uncertainty and paradox.
Practically, quantum theory enabled technologies from transistors and lasers to quantum computers and encryption. Philosophically, it posed challenges to our most basic assumptions about reality. Do particles have definite properties before we measure them? What role does observation play in determining the state of a system? These questions stretch beyond physics into the domains of philosophy and metaphysics, adding complex layers to humanity’s ongoing quest to comprehend the nature of reality.
In a profound sense, quantum mechanics demonstrated that our universe is not just stranger than we imagine; it’s stranger than we can imagine. Yet, it also offers the tantalising possibility that the fundamental laws governing the cosmos are within reach, waiting to be fully understood and integrated into a coherent picture of the universe.
As the 20th century drew to a close, and as we ventured further into the 21st, physicists found themselves at the threshold of perhaps the most ambitious project ever undertaken in theoretical physics: the unification of quantum mechanics and general relativity. The term ‘quantum gravity’ emerged as a placeholder for this dream, a theory that could describe the gravitational interactions at subatomic scales where both quantum mechanics and general relativity are relevant.
String theory emerged as one of the most promising candidates for such a unification. It postulates that the fundamental constituents of the universe are not point-like particles but tiny, vibrating strings. In this new framework, gravity is not a force mediated by a particle but a manifestation of the vibration of these strings. String theory also led to the startling conclusion that our universe may have more than the familiar three dimensions of space and one of time—a revelation that, if true, could rewrite the physics textbooks.
While string theory and its offshoots like M-theory offer a tantalising glimpse into a unified theory, they also bring new challenges. Experimental verification remains elusive, and the mathematics is incredibly complex. Yet, the mere possibility of unification offers a shimmering beacon to physicists and a profound statement to humanity as a whole.
The quest for unification, in a sense, represents the pinnacle of humanity’s ‘Search for Reality.’ It’s an endeavour to distil the cacophony of phenomena in our universe into a single, harmonious theory. It suggests that despite the bewildering complexity and vastness of the cosmos, there may be underlying simplicity and unity—qualities that have driven human inquiry since the dawn of time.
While the final chapter in this tale has yet to be written, the pursuit itself is a testament to human ingenuity and our relentless desire to decode the fabric of reality. It’s a quest that ties back to the philosophical musings of ancient civilisations, the ecclesiastical debates of medieval scholars, and the revolutionary scientific discoveries of the modern era. It’s a journey, with all its uncertainties, twists, and turns, that embodies the spirit of scientific naturalism—our unending endeavour to comprehend the universe through reason, observation, and experiment.
Our Present Understanding
in our ongoing ‘Search for Reality,’ it’s essential to highlight the twin pillars of contemporary scientific understanding: the Standard Model of Particle Physics and the Lambda-CDM model of Cosmology. These models serve as the culmination of centuries of scientific inquiry and technological progress, and they offer the most accurate descriptions of the universe at its smallest and largest scales, respectively.
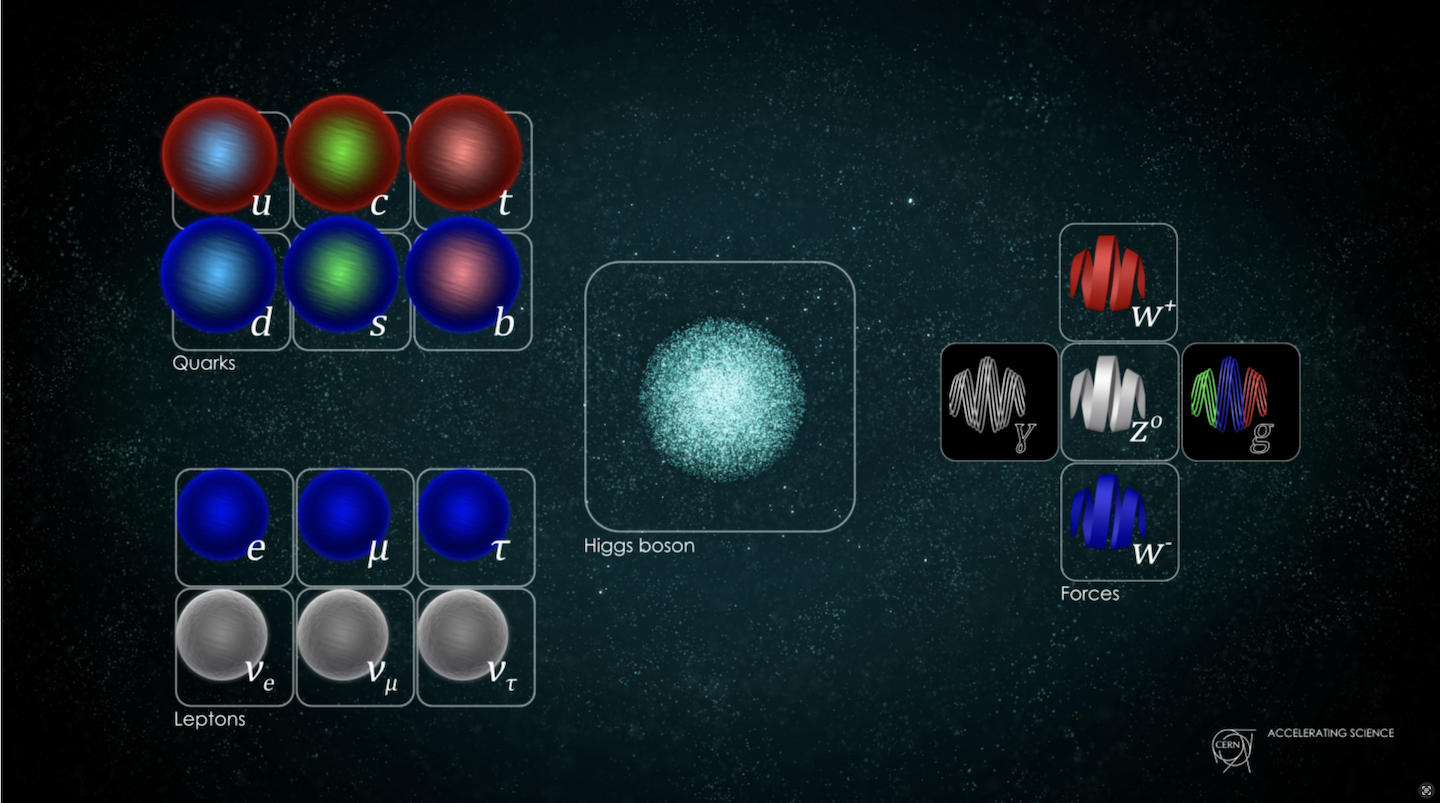
The Standard Model of Particle Physics is an incredibly successful theory that describes the electromagnetic, weak, and strong forces and the fundamental particles that make up the universe. This model has been confirmed through numerous experiments, most notably at particle accelerators like the Large Hadron Collider, where the last missing piece, the Higgs boson, was discovered in 2012. While extraordinarily successful, it leaves out gravity and thus is not a complete theory of everything.
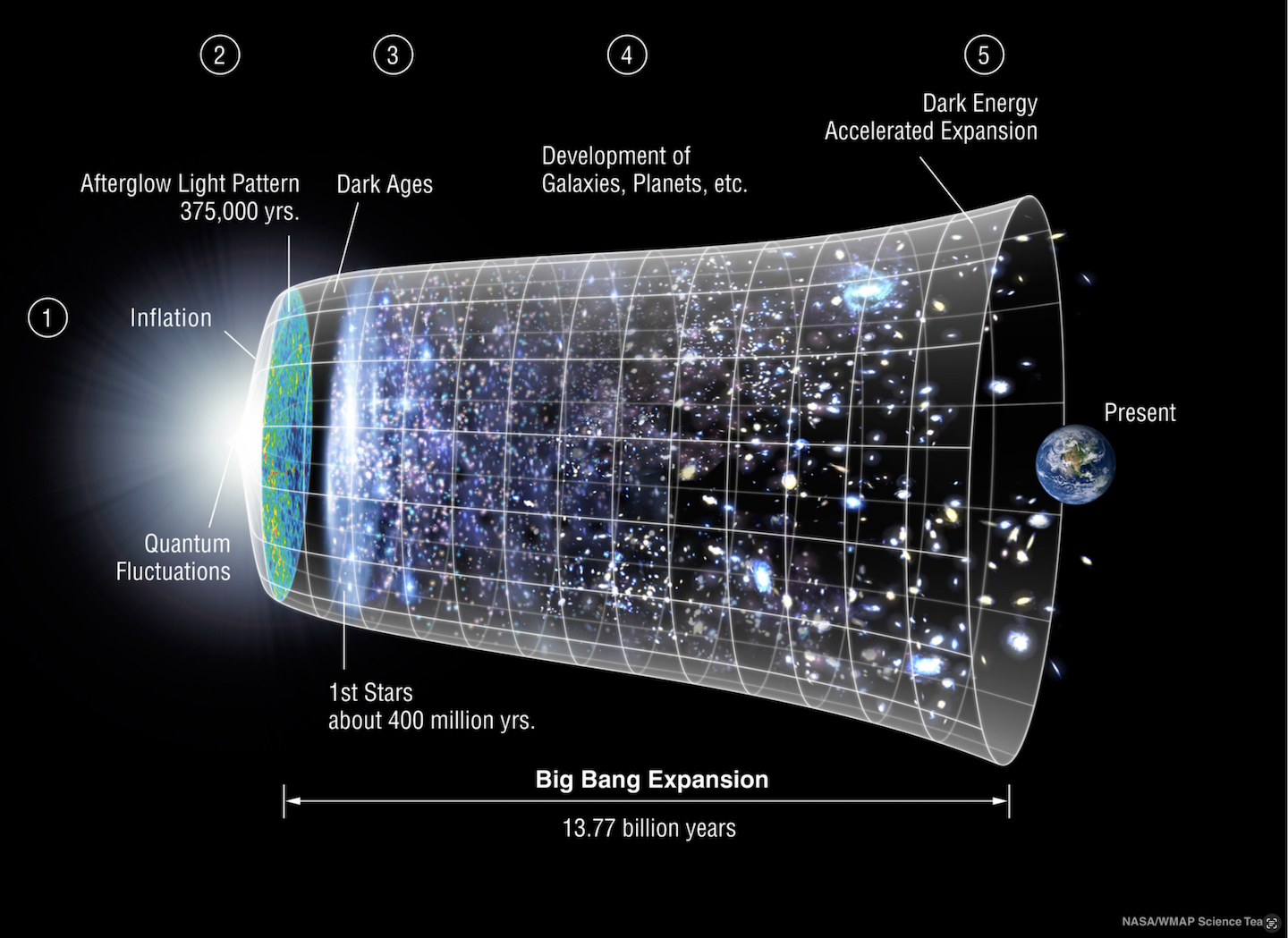
On the other end of the spectrum, the Lambda-CDM model describes the universe’s large-scale structure. It incorporates the presence of dark matter and dark energy. Observations of cosmic microwave background radiation, large-scale structure, and the distribution of galaxies support it. However, it also has its mysteries, most notably the nature of dark matter and dark energy, constituents that make up approximately 95% of the universe but remain elusive to direct detection.
Both models are extraordinarily successful but also marked by notable gaps. They describe a universe that is both understandable and profoundly mysterious. Each model excels within its own domain but fails to provide a unifying description that merges the quantum and cosmological realms. This is where theories like quantum gravity and string theory step in, seeking to bridge the divide and offer a unified description of reality.
Scientific Naturalism in Context
Standing on the shoulders of giants from the history of science, we find ourselves in a landscape rich with technological advancements and theoretical frameworks. Despite our monumental strides, however, it’s essential to recognise that scientific naturalism is one discourse among many seeking to explain the nature of reality. Philosophy, religion, the arts, and even the relatively nascent field of computational theory each offer different perspectives and methodologies.
The current scientific understanding—though incredibly robust and empirically grounded—still grapples with questions that lie on the fringes of the known. Dark matter, dark energy, the exact nature of consciousness, and even the foundational questions about the origins and eventual fate of the universe are areas where science has yet to provide conclusive answers. In the quest for a ‘Theory of Everything,’ we are reminded of the magnitude of what remains to be understood.
Yet, the value of scientific naturalism extends beyond its capacity for empirical verification. It serves as a framework that prioritises scepticism, requires evidence, and is willing to adapt when faced with new data. It’s a method more than a settled body of facts, emphasising the ‘how’ of understanding just as much as the ‘what.’
While science offers the most reliable means for understanding the physical universe, it coexists with other methods of inquiry that address aspects of reality that are less tangible but no less critical. Ethical imperatives, aesthetic judgments, and spiritual experiences are areas where science has limitations. Here, philosophy and spirituality offer complementary perspectives, enriching our collective discourse.
As we venture further into this complex tapestry of understanding, it becomes evident that each thread—be it scientific, philosophical, or spiritual—contributes to the richness and complexity of the human experience. None exists in isolation, and each informs the other in our endless quest to understand the most elusive of all concepts—reality itself.
Coda – A Personal Reflection
In this article, I briefly describe some of the pivotal steps in the development of scientific naturalism. I have not attempted to present a balanced discussion between different philosophical stances towards reality. I want to emphasise that this is not because I believe scientific naturalism to be the only valid perspective on “reality” – quite the contrary. While I believe in the power of the scientific method to understand the nature of the physical universe, I share many of the views of philosophers such as Raymond Tallis and Iain McGilchrist, who recognise the undeniable reality of the social world of relationships, ideas, and cultures that do not form part of the material universe but are created by humans and exercise significant influence over the part of the material world to which we have access – our earthly home.
As Dr Athena Potari writes, “Our world view shapes the ways in which we treat nature. The current model according to which matter, including nature, is seen as ‘dead’ and human beings as extrinsic to nature is a significant factor in the unprecedently destructive ways in which human beings have treated the natural world, leading to environmental hubris and corresponding ecological degradation.”
Terry Cooke-Davies
12 October 2023
This article was created with the assistance of ChatGPT Plus, and the original illustrations were created using Midjourney, an AI “text to graphics” programme. Illustrations of the Standard Models are from CERN and NASA respectively.